Want lower RF drift? Double down on laser PICs!
Integrating two lasers into a PIC device for satellite telecommunications generates radio frequency signals 10 times more stable than those generated by lasers on separate PICs. These results are the subject of a new paper in the Journal of Lightwave Technology, which compared the performance of TriPleX® external cavity lasers integrated on the same PIC to ones integrated on separate chips. Signal drift measured in the dual-laser PIC device is equal to 40 MHz, which translates to 10 times lower drift than that measured in lasers operating on separate PICs. This experiment was led by UC3M in partnership with LioniX under ThoRmux, a European Space Agency (ESA) funded project.
Satellite telecommunications (SatCom) is experiencing a need for larger bandwidth and more flexible payloads. This demand has created congestions in the traditional C and Ku frequency bands and a trend to move to higher frequencies in the microwave or millimeter-wave range, where there are plenty of available unexploited spectral resources. The main challenge with this approach is that the shift to higher frequency bands translates to increased size, weight, and power consumption (SWaP). Integrated Microwave Photonics (iMWP) technology provides a promising solution for overcoming this challenge by handling wide bandwidths, high frequencies, and spectral resources flexibility, all while maintaining low SWaP.
Within this frame, ThoRmux is an European Space Agency (ESA)-funded project that aims to develop a Tuneable Photonic RF Demultiplexer system with channel-flexible frequency allocation in the RF microwave domain, based on the TriPleX® iMWP technology. In this system, the photonic frequency generation unit is responsible for generating and distributing the RF local oscillator (LO) signal through optical heterodyning. In this technique, two optical frequencies (wavelengths) generated by two lasers are mixed in a photodiode to produce the RF LO signal with a frequency defined by the wavelength spacing of the lasers. UC3M, one of our project partners in ThoRmux, used the LioniX InP-TriPleX® dual-laser PIC module to generate RF LO signals in the microwave frequency band. Their deployment of the device highlighted clear advantages to integrating the two lasers on a single PIC. By comparing the stability of RF signals generated by two integrated lasers on the same PIC with those generated by two lasers on separate PICs, it was found that the RF signal had ten times lower drift with the first approach.
Figure 1: Diagram of the LioniX hybrid dual laser module used in the new JLT paper.
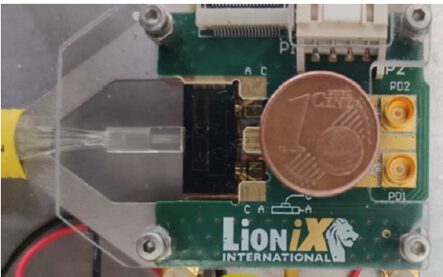
Fiber Array
TriPleX ® chip
2-PD chip
RSOA gain Section
RSOA gain Section
Figure 2: A photograph of the LioniX dual hybrid InP/Si3N4 laser module tested.
The InP-TriPleX® dual-laser PIC module
The functional design of the LioniX hybrid integrated dual laser module is shown in Figure 1. A picture of the fabricated assembly is shown in Figure 2. Each laser was made by the hybrid integration of an InP Reflective Semiconductor Optical Amplifier (RSOA) gain chip (represented as red boxes in Fig. 1) and an external cavity Si3N4 TriPleX® chip (represented as gray shaded areas in Fig. 1) via edge-to-edge coupling. The InP gain RSOAs have a highly reflective right side and an antireflective left side. The TriPleX® external cavity has two coupled ring resonators with slightly different Free Spectral Range (FSR) values to achieve single-frequency operation via the Vernier effect. In between the two mirrors, the lasers include a thermally adjustable Phase Tuning Section (PTS) and a 2×2 symmetric Mach-Zehnder Interferometer-based tunable coupler that allows control of the amount of light fed back to the laser cavity. The optical waveguide access ports are coupled to a Fiber Array. The TriPleX® waveguides offer low propagation loss of about 0.1 dB/cm, which enable Q-factors ranging from 20,000 to 3,000,000.
Experimental characterization of the Dual Laser PIC
Figure 3 shows the temporal evolution of the electrical drift for an RF signal at 34.6 GHz, generated by the optical heterodyning of LioniX dual-laser PIC device and measured over a period of 10 hours. The RF drift of the signal caused by the beating of the integrated lasers was around 30 MHz (as charted in Figure 3). Compared to optically heterodyning two lasers on separate PICS, this result indicates a 10 times improvement in RF drift. The improvement is attributed to the fact that the dual-laser chip uses a unique thermal controller, which ensures that the temperature compensation of thermal fluctuations on each laser are very similar to each other. The frequency shifts due to thermal control were thus correlated.
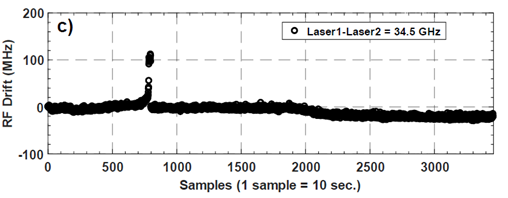
Figure 3: RF signal generation at 34.6 GHz from optical heterodyning between Laser 1 and Laser 2 of the LioniX dual-laser PIC assembly.
Further stabilization was investigated, using optical injection locking. The technique mitigates drift by locking the emission of one integrated laser using the second laser integrated on the same PIC. As a figure of merit, a locking range of 1.86 MHz has been reported. The experimental result is shown Figure 4.
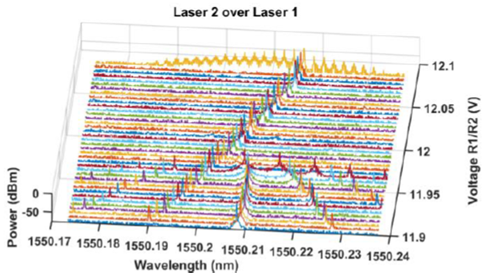
Figure 4: Optical spectrum of the injection locking experiment.
As Laser 2 approached Laser 1, it was observed an emergence of intermodulation products. This occurred due to Laser 2 being outside the locking range of Laser 1. The strength of the intermodulation products increased as the voltage applied to R1/R2 approached 11.96 V. Locking was achieved at 11.99 V, resulting in a clear signal. Laser 2 remained locked until the voltage reached 12.1 V, after which intermodulation phenomena reappeared. This signified that Laser 2 was no longer locked. A locking range of 1.86 GHz was attained, which is three times wider than the maximum reported drift during the initial attempt. This ensures stabilization of the laser even under adverse conditions.
The more, the merrier?
In future work, we aim to increase the number of on-chip lasers to 5 and develop an optical frequency comb by adding an integrated on-chip optical modulator. Figure 5 depicts the 3D model of the envisioned 5-laser PIC device. The integrated multi-carrier source allows center frequency flexibility for up to 4 RF channels, which is a huge advantage over conventional RF systems typically operating at fixed frequencies. Not only do these results show off the unique functionalities of integrated photonics and the flexibility of the TriPleX® platform, we envision opportunities for exploiting these results outside the satcom market.
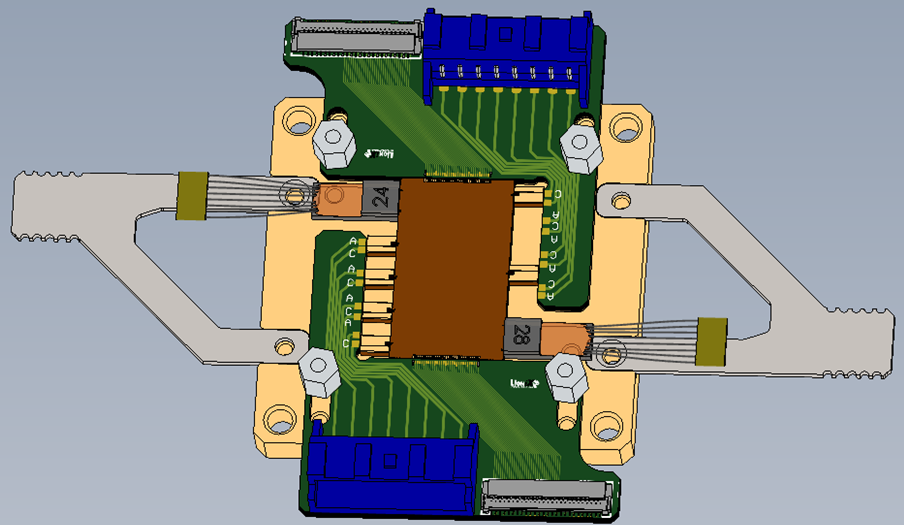
TriPleX® chip
6 InP Gain Sections (GSs): 1 dual-gain laser and 4 single-gain lasers
2 Fiber Arrays (FAs)
2 PCBs
51 pin connector allowing connection between heaters and driving electronics
XASK 2.5mm connector for the connection between GSs and driving electronics
Copper sub-mount
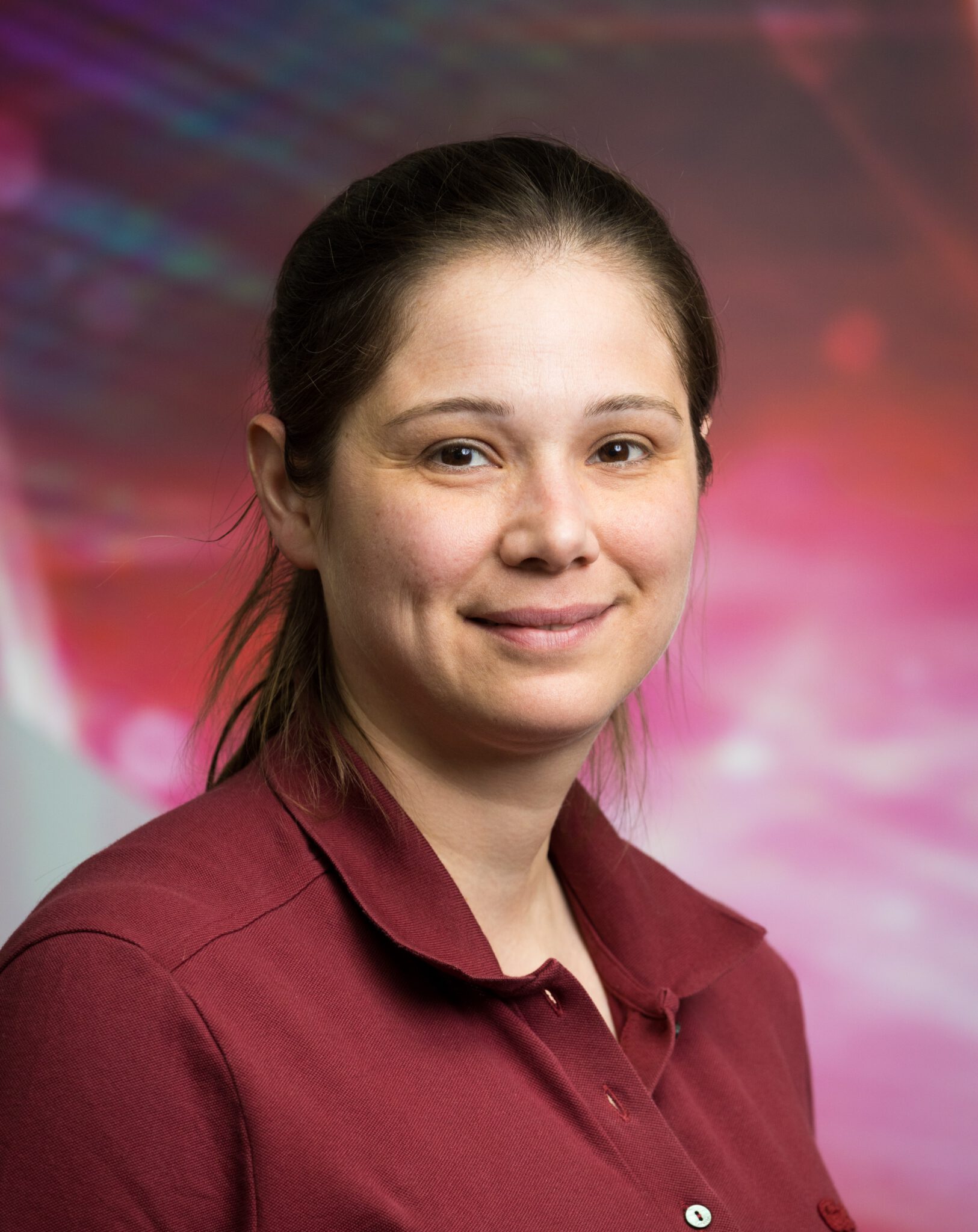
Dr. Charoula Mitsolidou obtained her Diploma degree from the Department of Electrical and Computer Engineering of Aristotle University of Thessaloniki in 2011 and her MSc degree in “Networks, Communication and System Architectures” from the Department of Informatics of the same university in 2013. In 2019, she received her PhD degree in “Radio-over-Fiber architectures for high throughput 5G networks” from the Department of Informatics of Aristotle University of Thessaloniki. Since 2020, she is working as a design engineer for microwave photonics systems in Lionix International.
Take a look at:
🌐 The academic paper in JLT by scientists in UC3M, SENER Aeroespacial, and LioniX International.
🌐An overview of integrated microwave photonics development at LioniX International.
🌐 Monthly updates on our developments and events via our newsletter!