Reconfigurable (DE)MUX Filter for Flexible Frequency Allocation
The growing demand for higher data rates, particularly with the rise of 6G and beyond, is putting significant strain on the limited available bandwidth in satellite communications. Traditional electronic filters, which process signals in fixed configurations, struggle to meet this demand efficiently. One path forward is through Microwave Photonics filters, which offer critical advantages over their electronic counterparts. These filters provide broadband tunability, reconfigurability, and the capability to achieve narrow fractional bandwidths, while also being resilient to electromagnetic interference (EMI). When integrated on a photonic chip, Microwave Photonics filters also deliver substantial improvements in Size, Weight, and Power (SWaP) consumption, enabling more flexible and capable systems in space.
Imagine satellite and telecom systems with the ability to reconfigure bandwidth and central frequency on demand, efficiently handling multiple channels without requiring numerous, single-purpose satellites. This vision is what the ThoRmux project, funded by the European Space Agency (ESA) and realized in collaboration with Sener Aerospace and UC3M aims to make a reality. Within this project, we are developing a Tunable Photonic RF Demultiplexer system that enables flexible frequency allocation across channels. Built on our ultra-low loss silicon nitride TriPleX® integrated microwave photonics (iMWP) technology, the heart of this system is the Photonic Integrated Circuit (PIC) (DE)MUX filter. This module provides the critical multiplexing and demultiplexing of multiple channels, with the flexibility to adjust bandwidth and central frequency dynamically.
Schematic representation of the (DE)MUX filter module
The released 4-channel DEMUX filter is based on the circuit topology shown in Figure 1. The 1-to-4 tunable optical splitter is responsible for splitting the incoming optical-converted 4-channel signal (f1, f2, f3, f4) to 4 independent and identical paths. Each output of the splitter is connected to an identical cascade of filters that form the channel selector. Specifically, the cascade of filters consist of 2 types of filters:
- The 1st type of filters is based on 8th order Coupled Ring resonator Optical Waveguide (CROW). This is the primary bandpass filter of the channel selector that serves as channel central frequency selector and BW regulator. The optical CROW filter has been designed for operation at the wavelength of 1550 nm and exhibits a Free Spectral Range (FSR) ≈ 2.5 GHz. LioniX Single Stripe waveguide technology has demonstrated optical losses lower than 1 dB/m, enabling the realization of Ring Resonator-based filters, such as the CROW filter, with extremely high quality factor (Q factor up to 300.000). The high-Q factors results on high resolution bandwidth and sharp roll-off filter response, which is highly attractive characteristics.
-
Figure 1: Schematic representation of the 4-channel (DE)MUX filter module
The 2nd type of filtering consists of 5th and 3rd order AMZI-lattice filters and serves as FSR extender. These filters are the secondary bandstop filtering stage that suppresses the 2nd and 3rd parasitic passbands of the CROW filter, extending the FSR of the channel selector to the value ≈ 10 GHz. The advantage of an AMZI-based filter is that it can be realized with a unlimited largeFSR, where ring resonator-based filters are limited by bending loss and length of a phase shifters in the resonator.
The (DE)MUX filter enables fully reconfigurable central frequency and channel BW in Ka, Q and V RF frequency bands. Reconfigurability is achieved by our novel technology of lead zirconate titanate (PZT) stress-optic actuators, benefiting from their very low power dissipation of ≈ 1 uW per actuator in quasi-static operation.
Produced 4-channel (DE)MUX PIC assembly
The produced module is presented in Figure 2. In the middle of the device is located the TriPleX® photonic wafer. In the middle of the wafer are placed the 1-to-4 splitter and the 4 “mandala”-shaped CROW filters, while around them are positioned the AMZI-based lattice filters. The optical inputs and outputs of the filters are interfaced to fiber arrays.
A total number of 318 stress-optic PZT-based tuning elements are employed to tune and reconfigure the various photonic filters of the device. The quasi-static power consumption per actuator is ≈ 1 uW, resulting on a total power consumption < 320 μW for the 4-channel PIC module. The electrodes of the PZT tuning elements are wire-bonded to customized Printed Circuit Boards (PCBs) with flat cable connectors that provide connectivity to the control electronics. The assembly is mounted on a copper sub-mount. The produced PIC assembly has a size of 129 mm × 135 mm × 5mm.
Figure 2: Produced 4-channel DEMUX PIC assembly
Simulated (DE)MUX filter response
Before the fabrication of the PIC module, we conducted a simulation study in order to estimate the spectral response of the filters. As shown in Figure 3, the analysis showed a tunable bandwidth ranging from 125 MHz to 1 GHz. The bandwidth reconfigurability is obtained by adjusting the power coupling coefficients of the tunable couplers between the 8 ring resonators of the CROW filter.
The central frequency tuning of each channel selector filters’ cascade is also demonstrated by simulations. By properly adjusting the tunable phase shifts of the CROW’s Ring Resonators and the Lattice’s AMZIs, we tuned the passband of each of the 4 channel selectors at a different central frequency. Figure 4 presents the case the 4 individual passbands are tuned to have a channel spacing of 2 GHz. Note that this is a case study example, while the channel spacing can be also reconfigurable.
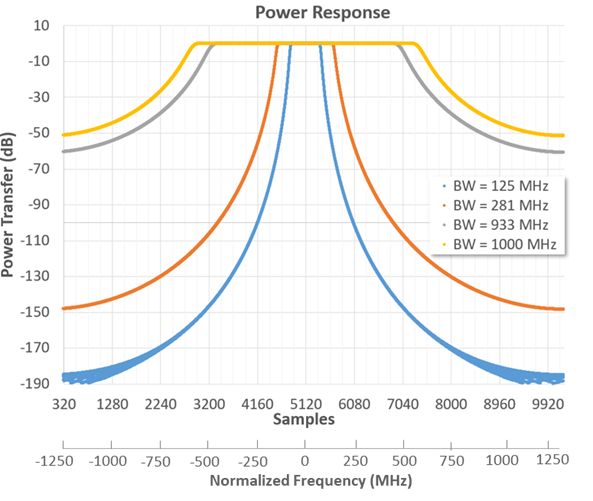
Figure 3: Simulated CROW filter power (left) responses for different passband widths
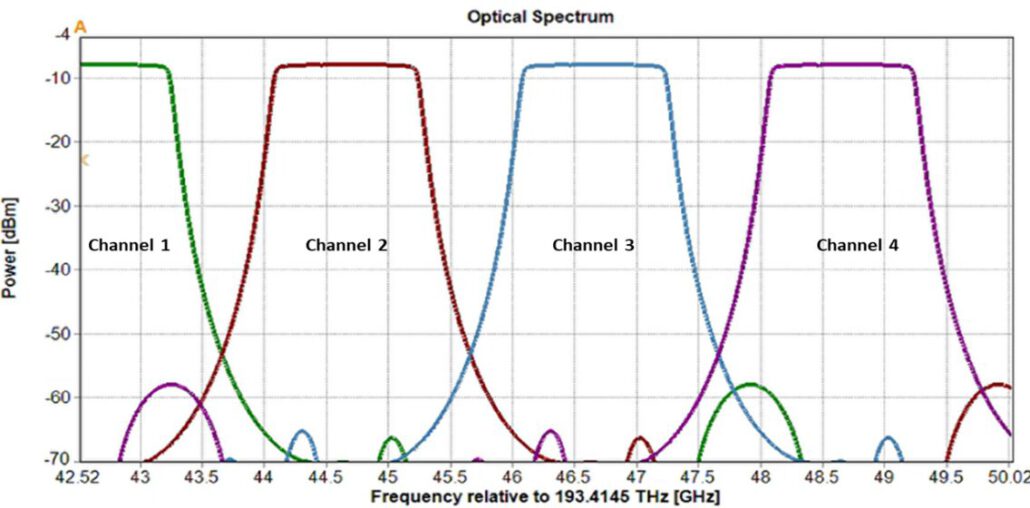
Figure 4: Simulated spectral response of the 4-channel DEMUX filter
The Future of Flexible Communication is just beginning
The results of our simulation of the filters were fully elaborated on in an earlier publication at the International Conference on Space Optics. We are now in the final stages of characterizing the PIC assembly. Results are very promising and closely align with the simulations. The full experimental analysis will be the subject of an upcoming talk at Photonics West 2025. We are excited to show you all that this device is capable of, and to continue leading iMWP developments in the future. Hope to see you in San Francisco!
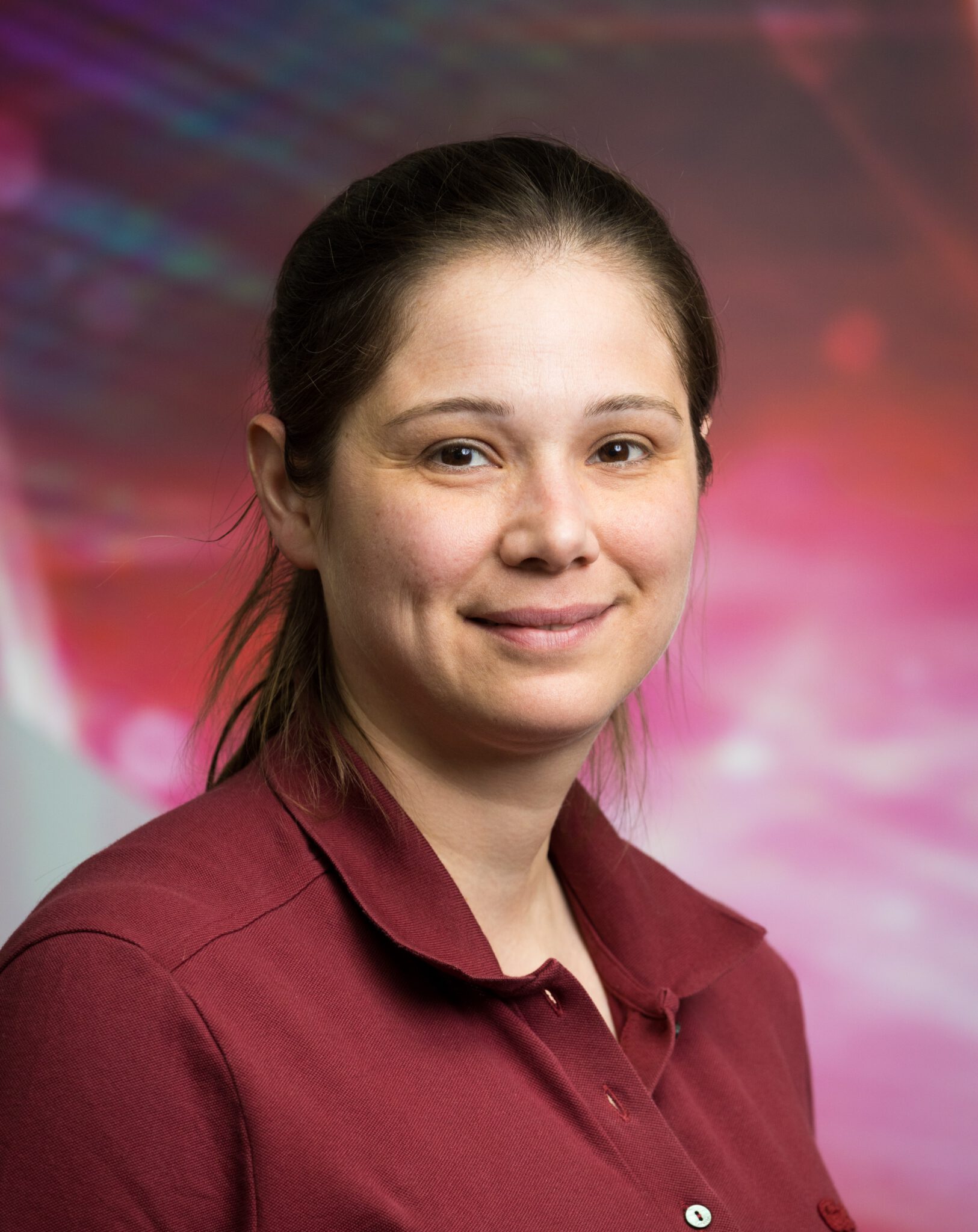
Dr. Charoula Mitsolidou obtained her Diploma degree from the Department of Electrical and Computer Engineering of Aristotle University of Thessaloniki in 2011 and her MSc degree in “Networks, Communication and System Architectures” from the Department of Informatics of the same university in 2013. In 2019, she received her PhD degree in “Radio-over-Fiber architectures for high throughput 5G networks” from the Department of Informatics of Aristotle University of Thessaloniki. Since 2020, she is working as a design engineer for microwave photonics systems in Lionix International.
Take a look at:
🌐Microwave photonics development at LioniX International.
🌐LioniX Multi Wafer Project (MPW) services.
🌐 Monthly updates on our developments and events via our newsletter!